Oxygen – a genomic caretaker
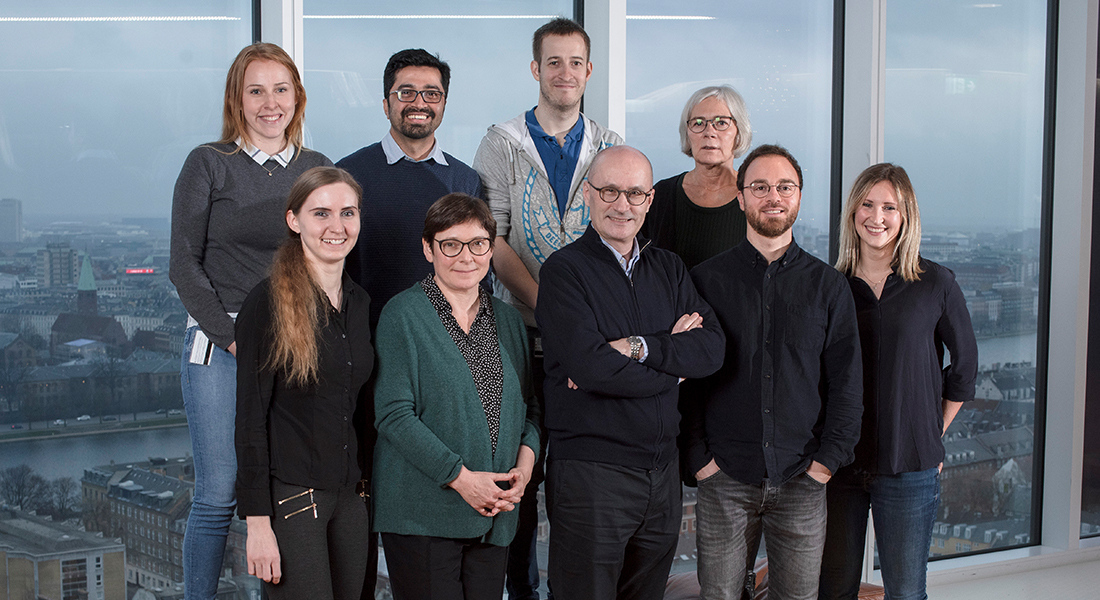
Oxygen is essential for almost all forms of life. Shortly after its accumulation in the atmosphere one billion years ago, organisms started to exploit molecular oxygen for energy generation. While this is still its principal biological function, oxygen-based molecules – known as reactive oxygen species (ROS) – gradually evolved into essential molecular messengers during cellular metabolism, stress reactions, immune responses, and injury-induced tissue regeneration. However, apart from supporting good things, too little oxygen or too much ROS can also do harm, for instance by driving the proliferation of cancer cells or by promoting neurodegeneration and aging. Thus, to foster desirable physiological effects and supress the harmful ones, most organisms including humans must constantly mobilize adaptive responses to maintain a perfect oxygen/ROS balance.
This can be best illustrated on cancer, where most developing tumors experience episodes of transient oxygen starvation (hypoxia) due to limited blood supply. This forces cancer cells to reprogram their metabolism and initiate the so called ‘hypoxia response’ in order to survive. Fundamental discoveries in this area of biomedicine were honored with the Nobel Prizes in Physiology or Medicine in 1931 to Otto Warburg and 2019 to Gregg Semenza, William Kaelin and Peter Ratcliffe. These pioneers and many other scientists established that the hypoxia response not only enables cancer cells to adapt to the hostile environment, but it also allows them to accumulate more mutations that further increase their proliferation and invasiveness. While this has long been recognized as crucial for cancer evolution, how exactly cancers fuel their mutation rates under low oxygen remained largely elusive despite it holds tremendous potential for a better understanding of the disease itself, as well as for designing new ways of cancer treatment.
In a multi-disciplinary research collaboration at the Novo Nordisk Foundation Center for Protein Research at the University of Copenhagen and the University of Texas at Austin, Kumar Somyajit, Julian Spies, and their colleagues from the Lukas, Mann, Jensen and Paull groups shed light on this fundamental biomedical question by connecting the three main elements of cancer progression into a molecular pathway: First, the researchers found that, facing oxygen starvation, cancer cells functionally suppress important DNA repair proteins involved in error-free, so-called homology-directed repair of damaged DNA. This results in an accumulation of unrepaired gaps of newly synthesized DNA (also called DNA-daughter-strand gaps) formed while cells duplicate their genomes. Second, they found that the same hypoxia that compromises DNA repair also liberates higher levels of ROS, which as a second messenger activates enzymes that further resects such unrepaired DNA daughter-strand gaps to the extend never seen in healthy cells. And third, the researchers noticed that such aberrantly extended DNA daughter-strand gaps are re-filled by highly mutagenic DNA polymerases, culminating into a rampant increase of mutation burden. Together, these findings provide thus far the most compelling mechanistic basis to understand accumulation of mutations in hypoxic tumors.
From a more general perspective, the new discovery can be interpreted such that the depletion of error-free DNA repair proteins transiently brings oxygen-starved cancers to a rather vulnerable stage, until they mobilize error-prone repair to accumulate sufficient combination of mutations that endows them with proliferation advantage. This is an important finding that could be potentially exploited to improve treatment. In other words, the time between the reduction of error-free repair and inception of the mutagenic one can be viewed as one of cancers’ ‘Achilles heels’ where a pharmacological intervention with the described mechanisms could be fatal for the incipient aggressive cancer.
Read more about the researchers' new results in the journal Developmental Cell: "Homology-directed repair protects the replicating genome from metabolic assaults"